Conversion of units
Paleomagnetic data contained in the old literature were reported in CGS-emu unit. Hence, it is necessary to convert the old data into SI unit if you want to compare them with new measurements. As the conversion of electromagnetic units is not as simple as those of length and mass, introduction to electromagnetic units for beginners is given here. Firstly it is pointed out that the magnetic field is often referred to \({\bf B}\), but this is the magnetic flux density (or magnetic induction), and is distinguished from \({\bf H}\) which is the magnetic field. Some of the SI units of magnetism are summarized as the followings.
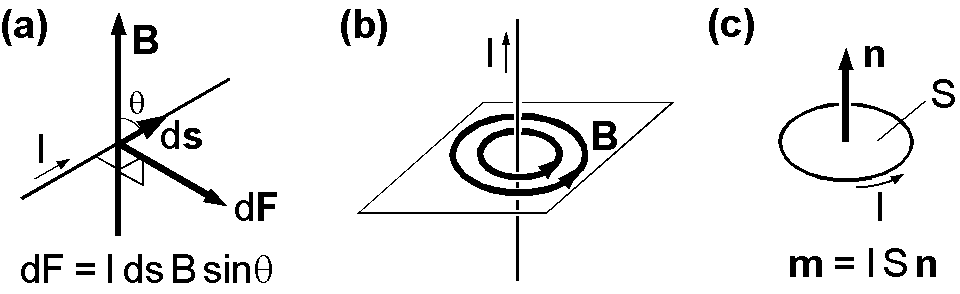
SI units of magnetism
Fig.(a) shows that the Lorentz force \({\bf dF}\) is acted on an electric current \(I\) flowing on the line element \(d{\bf s}\) which is placed in a magnetic flux density \({\bf B}\). This is formulated by the next equation in which the unit of \(I\) is ampere (A). \begin{equation} d{\bf F} = I d{\bf s} \times {\bf B}. \label{eq01} \end{equation} A force of 1 newton (N) per 1 m is created when \(I\) of 1 A is placed perpendicular to \({\bf B}\) of 1 tesla (T). Hence, the unit of \({\bf B}\), tesla, is expressed by more basic units as, \begin{equation} 1\ \mathrm{T} = 1\ \mathrm{N\,A^{-1}\,m^{-1}}. \label{eq02} \end{equation}
Fig.(b) shows that a current \(I\) flowing on a straight line produces a magnetic flux density \(B\) of circular form. Using the Biot-Savart law, it is shown that \({\bf B}\) is perpendicular to and concentric around \(I\), and its direction is clockwise when viewed toward the direction of \(I\). The strength of \(B\) is proportional to \(I\) and to inverse of the distance \(r\) from the current. This is formulated with a proportional constant μ0/2π as, \begin{equation} B = \frac{\mu_0}{2\pi}\frac{I}{r}. \label{eq03} \end{equation} The reason of introducing μ0 and 2π is a long story, and here suffice it to say that it will make the formulae of electromagnetism simple. Equations (1) and (3) indicate that two straight electric wires placed parallel will attract each other when the current is the same direction. When the currents are the same \(I\), the force \(dF\) acting on the line element \(ds\) of the wires is formulated as \[ dF = \frac{\mu_0}{2\pi}\frac{I^2}{r}ds, \] where \(r\) is the distance between the wires. With \(r\) = 1 m, the current \(I\) is defined as 1 A when the measured force is 2×10-7 N per 1 m of the wire. But this requires to assign the value of μ0 as, \begin{equation} \mu_0 = 4\pi\times 10^{-7}\ \mathrm{N/A^2}, \label{eq04} \end{equation} which is called the permeability of free space.
A magnetic moment \({\bf m}\) is defined as the loop current \(I\) multiplied by the area of the loop \(S\) as shown in Fig.(c), \begin{equation} {\bf m} = IS\,{\bf n}, \label{eq05} \end{equation} where \({\bf n}\) is a unit vector perpendicular to \(S\) and its direction follows the right-hand rule for the current direction. Hence, the unit of \({\bf m}\) is A m2. On the other hand, magnetization \({\bf M}\) is the total \({\bf m}\) contained within a unit volume, formulated with a small volume \(dv\) as \begin{equation} d{\bf m} = {\bf M}dv. \label{eq06} \end{equation} Hence, the unit of \({\bf M}\) is A/m. Lastly, the magnetic field \({\bf H}\) is defined as \begin{equation} {\bf H} = \frac{1}{\mu_0}{\bf B} - {\bf M}. \label{eq07} \end{equation} Hence, the unit of \({\bf H}\) is A/m, the same as \({\bf M}\).
Here, an expression of the unit tesla different from (2) is shown as the followings. According to the Faraday's law of electromagnetic induction, electromotive force \(\phi\) is induced in a circuit when the magnetic flux \(\Phi\) in the circuit varies as, \[ \phi = -\frac{d\Phi}{dt}, \] where \(\Phi\) is the product of \(B\) and the area of the circuit and its unit is weber (Wb) which is equal to \([\mathrm{tesla\times square\ meter}]\). Hence, the unit tesla is denoted with the unit volt (V) as, \begin{equation} 1\ \mathrm{T} = 1\ \mathrm{V\,s\,m^{-2}} \label{eq08} \end{equation}
Convert CGS-emu to SI
Now, convert \({\bf B}\) in CGS-emu unit to SI unit. Equation (1) also holds in CGS-emu unit in which the units of \(d{\bf F}\), \(I\) and \({\bf B}\) are dyn, abampere (1 abA = 10 A) and gauss (G), respectively. Hence, using (1), conversion of \({\bf B}\) is given by, \begin{equation} 1\ \mathrm{G} = \frac{1\ \mathrm{dyn}}{1\ \mathrm{abA}\times 1\ \mathrm{cm}} = \frac{10^{-5}\ \mathrm{N}}{10\ \mathrm{A}\times 10^{-2}\ \mathrm{m}} = 10^{-4}\ \mathrm{T}. \label{eq09} \end{equation}
Conversion of units for a magnetic field \({\bf H}\) and a magnetization \({\bf M}\) are obtained by comparing the next formulas. \begin{eqnarray} {\bf B} & = & \mu_0({\bf H} + {\bf M}) \quad (\mathrm{SI}), \label{eq10} \\ {\bf B} & = & {\bf H} + 4\pi{\bf M} \quad (\mathrm{CGS-emu}). \label{eq11} \end{eqnarray} Unit of \({\bf H}\) in CGS-emu is oersted (Oe) which is equal to gauss as known from (11). Hence, \({\bf H}\) of 1 Oe is equivalent to \({\bf B}\) of 1 G which is 10-4 T in SI unit according to (9). \({\bf H}\) in SI unit is obtained from (7) or (10) by dividing \({\bf B}\) by μ0 of (4). \begin{equation} 1\ \mathrm{Oe} = \frac{10^{-4}\ \mathrm{T}}{4\pi\times 10^{-7}\ \mathrm{N/A^2}} = \frac{10^3}{4\pi}\ \frac{\mathrm{N}}{\mathrm{A\,m}}\frac{\mathrm{A^2}}{\mathrm{N}} = \frac{10^3}{4\pi}\ \mathrm{A/m}. \label{eq12} \end{equation}
In CGS-emu, a magnetic moment \({\bf m}\) has a unit of emu (electromagnetic unit). As magnetization \({\bf M}\) is a total magnetic moment in a unit volume, its unit is emu/cm3. According to (11), \({\bf M}\) of 1 emu/cm3 produces \({\bf B}\) of 4π G which is, using (9), equivalent to 4π×10-4 T. Using (7) or (10), \({\bf M}\) in SI unit is obtained by dividing the last value by μ0. Hence, \begin{equation} 1\ \mathrm{emu/cm^3} = \frac{4\pi\times 10^{-4}\ \mathrm{T}}{4\pi\times 10^{-7}\ \mathrm{N/A^2}} = 10^3\ \mathrm{A/m}. \label{eq13} \end{equation} Using this relation, conversion of magnetic moment \({\bf m}\) is given by, \begin{equation} 1\ \mathrm{emu} = 1\ \mathrm{emu/cm^3}\times 1\ \mathrm{cm^3} = 10^3\ \mathrm{A/m}\times 10^{-6}\ \mathrm{m^3} = 10^{-3}\ \mathrm{A\,m^2}. \label{eq14} \end{equation} According to (11), unit of \({\bf M}\) in CGS-emu is also G and hence unit of \({\bf m}\) is also G cm3. But emu/cm3 and emu for \({\bf M}\) and \({\bf m}\), respectively, are often preferred to G and G cm3 to avoid a confusion with the unit G used for \({\bf B}\) in (9). In paleomagnetism, magnetic moment per unit mass is often used. Using (14), conversion of the units is, \begin{equation} 1\ \mathrm{emu/g} = \frac{10^{-3}\ \mathrm{A\,m^2}}{10^{-3}\ \mathrm{kg}} = 1\ \mathrm{A\,m^2/kg}. \label{eq15} \end{equation}
Magnetic susceptibility \(\chi\) (dimensionless) is defined by the next equation for both SI and CGS-emu units. \begin{equation} {\bf M} = \chi {\bf H}. \label{eq16} \end{equation} Suppose \({\bf H}\) of 1 Oe induced \({\bf M}\) of 1 emu/cm3, \(\chi\) is 1 in CGS-emu unit, which is converted to SI unit as, \begin{equation} 1\ _{\mathrm{CGS}} = \frac{1\ \mathrm{emu/cm^3}}{1\ \mathrm{Oe}} = \frac{10^3\ \mathrm{A/m}}{10^3/4\pi\ \mathrm{A/m}} = 4\pi\ _{\mathrm{SI}}. \label{eq17} \end{equation} Mass specific susceptibility is obtained by dividing the above volumetric susceptibility by density. Hence, conversion of CGS-emu to SI is given by, \begin{equation} 1\ _{\mathrm{CGS\,mass}}\ \mathrm{cm^3/g} = \frac{1\ _{\mathrm{CGS}}}{1\ \mathrm{g/cm^3}} = \frac{4\pi\ _{\mathrm{SI}}}{10^3\ \mathrm{kg/m^3}} = \frac{4\pi}{10^3}\ _{\mathrm{SI\,mass}}\ \mathrm{m^3/kg}. \label{eq18} \end{equation}
Summary of units conversion and an example of application are given in the followings.
Quantity | CGS-emu | SI |
Magnetic flux density \({\bf B}\) | 1 G | 10-4 T |
Magnetic field \({\bf H}\) | 1 Oe | 103/4π A/m |
Magnetization \({\bf M}\) | 1 emu/cm3 (G) | 103 A/m |
Magnetic moment \({\bf m}\) | 1 emu (G cm3) | 10-3 A m2 |
Magnetization (mass specific) | 1 emu/g | 1 A m2/kg |
Magnetic susceptibility \(\chi\) | 1 | 4π |
Magnetic susceptibility (mass specific) | 1 cm3/g | 4π/103 m3/kg |
Example of conversion of magnetic units
Suppose we found a past report in CGS-emu on an intensity of NRM (natural remanent magnetization), a \(Q_n\)-ratio (a ratio of NRM to an induced magnetization under the present field), and stability to AF (alternating field) demagnetization.
\(M\) was 5×10-3 emu/cm3 and \(\chi\) was 2×10-4. Hence, \[ Q_n = \frac{M}{\chi H_0} = \frac{5\times 10^{-3}\ \mathrm{emu/cm^3}}{2\times 10^{-4}\times 0.5\ \mathrm{Oe}} = 50, \] suggesting high stability of NRM which remained up to magnetic field of 1000 Oe in AF demagnetization.This is rephrased in SI unit as,
\(M\) was 5 A/m and \(\chi\) was 8π×10-4. Hence, \[ Q_n = \frac{M}{\chi H_0} = \frac{5\ \mathrm{A/m}}{8\pi\times 10^{-4}\times 0.5\times 10^3/4\pi\ \mathrm{A/m}} = 50, \] suggesting high stability of NRM which remained up to magnetic field of 100 mT in AF demagnetization.Please note that the "100 mT (millitesla)" in the last sentence is not correct which should be "106/4π A/m". However, in paleo- and rock magnetism, \({\bf H}\) is often referred to \({\bf B}\) because without a tedious factor of 4π it is much easier to compare the data between SI and CGS-emu.
Note:
Following the above mentioned convention of paleo- and rock magnetism, in this home page, the values of magnetic field \({\bf H}\) will often be given in the unit of tesla (T).